

Department of Geological Sciences
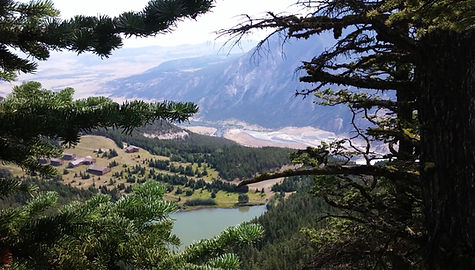
Dr. Adriana Heimann Ríos
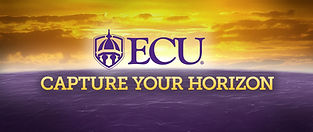

Mineralogy, Petrology, Geochemistry & Economic Geology Research
General Overview
Fundamental and applied research is focused on understanding igneous, sedimentary, metamorphic, and hydrothermal processes responsible for the origin and evolution of rocks and their economic mineral deposits using mineralogy, petrology, geochemistry, stable isotope geochemistry, and modeling. Part of my expertise is specifically in the geochemistry of magmatic, hydrothermal, and metamorphic rocks and associated mineral deposits of strategic rare metal (Li, Ta, Be), Fe, and base metal sulfide deposits. To solve problems, I use detailed mineral and whole-rock major-, trace-element and isotope geochemistry (Fe, C, O), and state-of-the-art analytical techniques (electron microprobe, SEM-EDS, LA-ICP-MS, Raman spectroscopy, synchrotron radiation (XAS, XRF), MC-ICP-MS, and age dating, among others). Petrographic analysis, clean laboratory techniques (sample dissolution, ion exchange chromatography), theoretical and numerical modeling, and statistical analysis are common tools. My field work so far takes place in North America, South America, and Australia. I have connections with scientists, industry, private and government exploration and mining companies, and other organizations in Australia, Uruguay, the U.S., and Canada, and new collaborations are always welcome. A new collaborative project, our LIT GEO project, focuses on a dream passion: community-based and societal relevant geoscience working alongside community organizations in North Carolina and Puerto Rico.
Some Current Research Projects
Iron Isotope Fractionation in Magmatic Systems. Overview
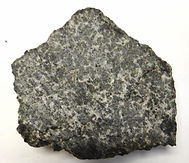
Gabbroic rock from Skaergaard. Rocks were kindly provided on loan by Dr. Alan Boudreau (Duke University).
The fractionation of stable iron isotopes in terrestrial systems and other planetary bodies continues to be the subject of considerable study and debate after initial developments of the Fe isotope system in the early 2000s. Tens of highly visible research articles continue to be published on top scientific journals every year on the subject of Fe isotope fractionation in igneous and metamorphic rocks, crustal and mantle processes on Earth and extraterrestrial bodies, and their application to understanding the genesis and characteristics of ore deposits. There is need and potential for further development, especially due to continuous improvements in mass spectrometry. For example, the origin and significance of inter-mineral Fe isotope fractionation and overall fractionation in terrestrial and extraterrestrial rocks are yet to be agreed upon within the scientific community. Because deciphering the extent, if any, of Fe isotope fractionation in igneous rocks during fractional crystallization answers bigger questions of fractionation during planetary formation processes in our solar system, these problems will continue to be investigated. Part of my current research is on elucidating the causes of Fe isotope fractionation in magmatic and hydrothermal processes and my plan is to continue investigating the role of various factors in fractionating Fe isotopes.
M.S. students Allison Murrie, Brett Pertunen, Tiiffany Cummings, and Alex Hammerstrom worked on this project that focuses on the Skaergaard layered mafic Intrusion, Greenland. This projects is in collaboration with Drs. Clark Johnson, Brian Beard, and John Valley (UW-Madison). Funding from NSF Petrology and Geochemistry, Heimann-Rios PI.
Specific Problems in Fe Isotope Geochemistry
The extent and causes of Fe isotope fractionation during magma evolution and hydrothermal alteration in layered-mafic intrusions.
This project is a new direction developed as an assistant professor and funded by NSF (Heimann-Rios, P.I.) that extends from post-doctoral investigations of the novel Fe isotope system in igneous rocks. My postdoctoral research at the University of Wisconsin, Madison, with the pioneer of Fe isotope geochemistry, Dr. Clark Johnson, focused on understanding the mechanism of fractionation in high-silica igneous rocks that recorded a larger fractionation than in mafic-intermediate rocks by using a combination of mineralogy, geochemistry, and modeling. To explain the fractionation of Fe isotopes, I proposed a new mechanism of fluid/rock interaction, in contrast to previously suggested fractional crystallization (Heimann et al. 2008). Current independent work with graduate students includes investigating the extent and significance of inter-mineral, bulk-mineral, and bulk-rock Fe (and O) isotope fractionation in Fe-Ti oxides, bulk-rock gabbroic rocks, and Fe silicates during magma evolution and hydrothermal alteration throughout the well-studied Skaergaard layered mafic intrusion, Greenland. Based on theoretical and experimental considerations, Fe isotope fractionation between magnetite and Fe silicates at crystallization temperatures should be small (~ 0.16‰; Fig. 1), but previous studies reported larger inter-mineral Fe isotope fractionation values and Fe isotope values (Fig. 2). We obtained successful results via a detailed mineralogical, mineral chemistry, major-, trace-element and O isotope geochemistry, and modeling study that yields new insights into the nature (equilibrium vs. non-equilibrium; fractional crystallization, alteration) of Fe isotope fractionation in this system that can be applied to understand other terrestrial systems and crustal formation on Mars, the Moon, and other planetary bodies. Significant advances were made to explain the measured variations in dual-valence Fe-Ti oxides, which had yet to be fully understood, as well as in fresh and altered rocks (Heimann et al. In Prep., Heimann et al. 2016, 2020, Hammerstrom et al. 2016, 2020, 4 M.S. theses). Iron isotope data were obtained over two weeks by the PI and two graduate students at the Fe isotope lab of Dr. Clark Johnson at UW-Madison in collaboration with research scientists, Dr. Johnson, and Dr. Brian Beard. Oxygen isotope compositions of minerals and rocks were obtained in collaboration with Drs. John Valley and Spicuzza at UW-Madison. In addition, in collaboration with Drs. John Valley and Noriko Kita at UW-Madison, we are investigating the fractionation of oxygen isotopes in individual crystals from the Skaergaard intrusion using in-situ SIMS analysis to understand planetary formation processes (Zhang et al. 2022). We are also collaborating to create new standards for O isotope analysis of silicates with chemical compositions for which standards are not yet available.

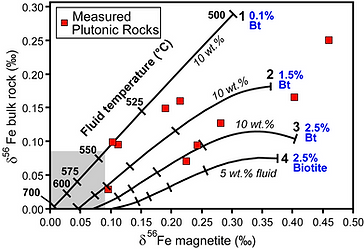
Figure 2. Modeled magnetite and bulk-rock d56Fe values during fluid exsolution. From Heimann et al. (2008).
Origin and Significance of Precambrian BIFs for Early Earth Environments. Overview

Another area of inquiry with a large number of yet to be answered questions in which I am currently working is the occurrence, geochemistry, origin, and significance of Precambrian banded iron formations (BIFs) on the terrestrial Archean and Proterozoic rock record for understanding the early Earth’s biosphere-atmosphere-solid Earth system, as well as the role of bacteria during their formation. These have been the continuous focus of detailed studies for at least the last 70 years. Yet, models for their formation, demise, and brief reappearance during the Neoproterozoic snowball Earth are currently debated. Studies are dedicated to determine Fe(II)-Fe(III) redox reactions that formed oxidized and reduced mineral phases, identifying bacterial biosignatures in BIFs that might be used in the search for early life on other planets, correlations of coeval sequences in distant locations and previously unstudied sequences that can inform of global vs. local conditions of formation and disappearance, and the redox conditions in early oceans recorded in these ancient marine chemical precipitates, among others. I have been working on the geochemistry of BIFs and its significance for early Earth environments since I was an undergraduate student and plan on continuing this research area.
Photo showing mesoscopic layering in BIF from the southwestern Superior Province, Ontario, Canada.
Red = jasper, white = quartz, dark = hematite/magnetite
Specific Problems on the Geochemistry and Genesis of BIFs
The study of the Fe isotope composition of BIFs in the rock record is largely incomplete, particularly of those with ages around the time when BIFs became less abundant, at < 2.2 Ga (Fig. 3). One of the objectives of my research is to continue filling the gaps in the record through time of Fe isotope composition of marine chemical precipitates to determine if the variations currently observed with a negative excursion at ~ 2.5 Ga and an increase in values after that is actually related to the expansion of dissimilatory iron reducing (DIR) bacteria at that time or just the result of an incomplete record. The goal is to determine if various BIF sequences around the world indeed record the physicochemical characteristics and evolution of early Earth’s oceans or purely diagenetic processes related to cycling of Fe by DIR bacteria, and to identify, if possible, biosignatures indicative of early life on Earth for the search of early life on other planets. This research is an independent extension of a prior project conducted with Drs. Johnson, Beard, Valley, and Roden (Heimann et al. 2010) that focused on the origin of the ~2.5 Ga Kuruman Iron Formation in which I used detailed carbonate mineralogy, mineral chemistry, combined Fe-C-O isotope compositions of minerals, and theoretical modeling to propose a new mechanism for the formation of marine Fe carbonates prior to the Great Oxidation Event via redox Fe cycling by dissimilatory iron reducing (DIR) bacteria during diagenesis at the water-sediment interface (Heimann et al. 2010). My finding of micro-scale hematite inclusions within Fe carbonates was fundamental to explain the formation of Fe(II) carbonates via Fe(III) reduction by DIR (Fig. 4). This study led to a further study and a highly visible publication that concluded that the combined geochemistry of BIFs reflects diagenetic processes, and not marine redox conditions (Johnson et al. 2013). Recent and current projects funded so far by the NASA North Carolina Space Grant (Heimann-Rios, P.I.) expand the geographic sampling and representability of previous BIF studies by focusing on less known ~2.5 Ga BIFs from South America and 1.69 Ga IFs from Australia formed at critical times in Earth’s history in locations away from the well-studied Kaapvaal-Hamersley basin. The data obtained so far by M.S. and undergraduate students, including mineral chemistry, whole-rock major- and trace-element geochemistry, rare earth elements (REEs), and U-Pb age dating (Rosière et al. 2019, Lancaster et al. 2014, Serna et al. 2013), will be expanded to incorporate Fe-O isotope compositions and used to investigate and decipher whether variations in Fe-O isotope compositions of minerals reflect Fe3+/Fe2+ ratios, diagenetic cycling of Fe by bacterial DIR, and/or the redox state of the oceans in new locations. An important focus of these BIF studies is the REE composition of the Precambrian oceans and making comparisons across the globe (e.g., Heimann et al. 2010, Rosière et al. 2019). Similarly, a study of ~1.69 Ga BIFs associated with late Paleoproterozoic marine hydrothermal sulfide deposits near the giant Pb-Zn Broken Hill deposit, Australia, and comparisons with similar IFs from other localities that formed at about the time when large late Paleoproterozoic BIFs ceased to appear in the rock record, provides the ground work to further investigate the Fe isotope composition and ultimately determine if these IFs record the same physicochemical characteristics (fO2, chemical composition of fluids, bacterial cycling of Fe-C) worldwide. These BIF studies will be the subject of future NSF and NASA proposals.
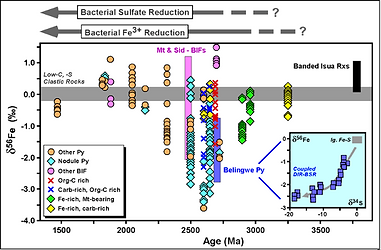
Figure 3. Iron isotope record of marine sedimentary rocks, including BIFs, through time. From Johnson et al. (2008).
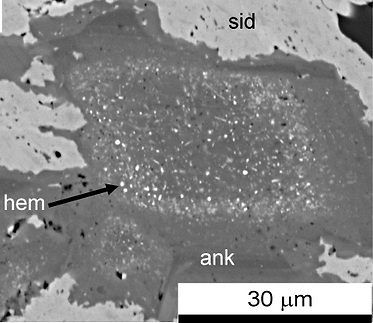
Figure 4. Scanning electron microscope back scattered electron image from the ~2.5 Ga Kuruman Iron Formation, South Africa, showing the occurrence of Fe oxide (hematite) inclusions in ankerite (Ank). From Heimann et al. (2010).
My new independent work resulted in two book chapters on BIFs (Rosière et al. 2019, Heimann 2021) and several manuscripts being finalized based on graduate and undergraduate student’s theses. Understanding bio-physicochemical conditions in the early Earth marine record by mineralogy and isotope geochemistry studies of BIFs is a change in direction and expansion to more fundamental questions from graduate study investigations. In the latter, I studied the geochemistry of metamorphosed marine chemical precipitates and hydrothermally altered rocks associated with marine Proterozoic massive sulfide deposits in Colorado and Australia to determine the physicochemical conditions of formation using major- and trace-element and isotope geochemistry (Heimann et al. 2005, 2006, 2009, 2010, 2013).
Near-future projects to expand the record through time of investigated BIFs will focus on Cryogenian (Neoproterozoic Snowball Earth) BIFs, including some almost unstudied BIFs from the Appalachian Mountains and Uruguay, iron formation associated with the marine hydrothermal Broken Hill deposit, Australia, and a comparison of North and South American BIFs to determine if they reflect global or local patterns, which is still little known. For several little studied BIFs, we have mineral chemistry and bulk-rock major- and trace-element chemical compositions that will be complemented with Fe-O isotope data to interpret the genesis of these critical rocks to understand early Earth marine environments.
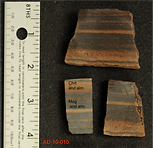
Example of project: Mineralogy, petrology, geochemistry, and genesis of iron formations near the giant Broken Hill Pb-Zn-Ag deposit, Australia. This is a collaboration with Dr. Paul Spry (Iowa State University). MS student Erica Serna worked on this project. Funded by the North Carolina Space Grant (NASA), Sigma Xi, and East Carolina University.
Physics and Chemistry of Minerals to Explain Crust Formation Processes. Overview
The advancement of new, state-of-the-art synchrotron radiation techniques such as X-ray absorption spectroscopy (XAS) and micro-XRF and Raman spectroscopy, among others, are allowing to better constrain the nature, abundance, and structural environment of transition elements such as Fe, Cr, and Mn, the determination of the concentration of Fe and Fe3+/Fe2+ ratios, and their distribution within crystals at the sub-micrometric scale with a level of detail not possible until recently. These parameters can then be used to understand a variety of phenomena and processes, including the origin of color in minerals and particularly those that form economically important, gem-quality minerals or critical mineral deposits. Similarly, determination of OH and other volatiles in nominally anhydrous minerals by Raman spectroscopy combined with FTIR are allowing the in-situ characterization of fluids and volatiles in minerals that can then be used to fingerprint the chemistry of magmas and fluids and apply them to understand their evolution or characterize mineralizing systems. However, standards have yet to be developed for XAS analysis of many minerals, and polarization effects have to be determined for XAS and Raman spectroscopy techniques before these tools can be effectively used for further developments. Therefore, we are currently doing developmental work to understand polarization effects in synchrotron-based XAS and XRF spectroscopy and Raman spectroscopy analysis. Developing these techniques will allow their use in other areas of study, including analyzing materials on other planets by rover missions to determine the presence of water.
Specific Problems on the Physics and Chemistry of Minerals to Determine Crust Formation Processes
1) Quantification of the valence state and structural role of transition metals (Fe, Mn, Cr) within minerals to explain formation processes in a variety of settings.
This is a new research direction started within the last three years currently funded by an internal research grant and a project approved by the Advanced Photon Source (APS) of Argonne National Lab to characterize minerals using state-of-the-art analytical techniques (synchrotron radiation micro-XAS and XRF, Raman spectroscopy). We are particularly focusing on Fe due to its dual oxidation state, which can be used to decipher differences in redox conditions during formation processes from magma and hydrothermal fluids. We are first laying the groundwork to explain polarization effects in XAS and Raman analyses, which need to be understood before data can be used accurately to make interpretations (Gray et al. 2019). Combined micro-XAS and micro-XRF data obtained so far show sub-micrometric scale elemental zoning and variations in the structural role of Fe within crystals that can be used to understand the evolutional changes of fluids and magmas during crustal formation processes (Tomasic et al. 2021). We are using X-ray Larch/Python modeling of the extended X-ray absorption fine structure area of XAS Fe K-edge spectra to determine bond lengths (Fe-O, Fe-Fe) and variations related to the coordination geometry and valence state of Fe, which can be used to determine variations in the redox state during mineral formation (Fig. 5). Part of project, in collaboration with Dr. Alonso-Perez from Harvard University, is to explain the role of variations in valence state and coordination geometry of chromophore elements to explain the origin of color in mineral species. Advancing this research requires development of standards for quantification of Fe3+/Fe2+ ratios, which involves incorporating additional analytical techniques, particularly Mössbauer spectroscopy and photoluminescence spectroscopy, to measure Fe3+/Fe2+ ratios in the same minerals analyzed by XAS to be used as standards to then quantify unknown Fe3+/Fe2+ ratios in minerals. This is the subject of an NSF proposal under preparation using preliminary XAS and XRF data obtained at the Advanced Photon Source and Raman spectroscopy data obtained at Harvard University in which I am collaborating with physicists and other scientists (Alonso-Perez et al. 2018, Gray et al. 2019).

Figure 5. Example of a graph showing the Fourier spectra fitting of EXAFS Fe K-edge data in a beryl crystal used to determine bond lengths and coordination. Heimann, Unpub. data, In Prep.
2) In-situ identification of water/OH and other volatiles in nominally anhydrous minerals.
This is a new project and overall new area of research that aims at identifying genetic differences in fluid and volatile composition in felsic rocks and veins with economic vs. uneconomic critical mineral (Li, Be) deposits and ultimately identify differences in fluid/volatile composition in different geologic environments. Raman spectroscopy is being used to analyze samples in situ in a non-destructive way to identify the “type” of water molecules present in various sites within the mineral structure as well as other volatiles such as CO2, CH4, etc. (Fig. 6). This first part of the project was funded by an internal research initiation grant and is being conducted in collaboration with Dr. Alonso-Perez from Harvard University. with whom we are advising graduate students and we count with the opportunity to take them to Harvard University to conduct Raman spectroscopy analyses (Gray et al. 2019). Preliminary Raman spectroscopy data on different minerals are promising to determine the presence and type of water and identifying differences between different geologic settings, but the results need to be calibrated using FTIR, which will be the next steps in the project, and this is the focus of an NSF proposal in preparation (Heimann-Rios, PI). In the near-future, my goal is to study and characterize water in minerals and identifying differences in the water content, budgets, and cycling between the terrestrial and Martian crust and mantle. This is the subject of a NASA proposal in preparation in which I am collaborating with several scientists, particularly from the NASA Johnson Space Center.
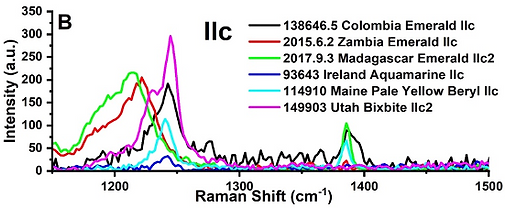
Figure 6. Raman spectra of beryl exhibiting carbon dioxide peaks at ~1240 cm-1 and ~1380 cm-1 in a crystal. Beam oriented parallel to the crystal's c axis. Gray et al. 2019, Heimann, Unpubl.
3) Origin, elemental behavior, and chemical evolution of felsic magmatic melts which can lead to the formation of strategic rare metal (Li, Be, Ta, REEs) mineralization or barren felsic dikes and granitic pegmatites.
Recent and current research funded by the U.S.G.S. Mineral Resources External Research Program is using detailed in-situ major- and trace-element chemical compositions of accessory minerals (obtained by LA-ICP-MS) in different kinds of felsic dikes worldwide (Heimann 2013, Heimann et al. 2015, 2016, Mitchell & Heimann 2017) to explain the behavior of several elements, including some not traditionally investigated (Zn, Mn, Cr), during chemical evolution and crystallization of felsic magmatic melts and for which little is known. We are using multivariate statistical analysis to distinguish chemical and geologic setting differences (host rock, emplacement depth, age, deformation, metamorphism) to define new mineral chemical petrogenetic indicators that reflect physico-chemical conditions of formation and likely source of melts (igneous S-, I-, or A-type vs. sedimentary rocks) (Mitchell & Heimann 2017). My group obtained the first in-situ trace-element compositions of gahnite ([Zn,Fe,Mg]Al2O3) in various kinds of felsic dikes (Yonts et al. 2013, 2014, Heimann et al. 2015, 2016), and we are using the mineral chemistry of several minerals to understand the chemical characteristics of different granitic pegmatites and the overall characteristics of melts that produce rare-metal deposits or barren rocks. We are also identifying mineral chemistry differences between felsic dikes and metamorphic rocks to quantify chemical parameters that distinguish the two types of rocks and element behavior during magma evolution and metamorphism. This is the subject of an NSF proposal to be re-submitted (Heimann-Rios P.I.). This research diversified from early graduate studies in which I defined mineral chemistry parameters indicative of metamorphosed massive sulfide deposits vs. other rocks based on the major- and trace-element composition of accessory minerals (Heimann et al. 2005, 2011). This research also evolved from an early project undertaken with a post-doctoral researcher in which we studied the major- and trace-element chemistry of colored beryl varieties from granitic pegmatites in Argentina to determine the relative fractionation of felsic melts and elemental behavior during crystallization, for which in 2015 we received the Hawley Medal for the best paper in The Canadian Mineralogist (Sardi & Heimann 2014).
Characterizing beryl varieties with state-of-the-art analytical techniques: This is a relatively new project with colleagues Dr. Raquel Alonso-Perez, Tony Lanzirotti, and Darby Dyar. One of the goals is to understand the behavior of strategic elements (Li and Be) in felsic melts that form granitic pegmatites with economic mineralization or barren rocks.
Stay tuned for other details. Funding from a Research Initiation Grant (Arts and Sciences, REDE). Daniel Gray and and Brandon Nickerson worked on this project and current M.S. student Patrick Tomasic is working on the project.
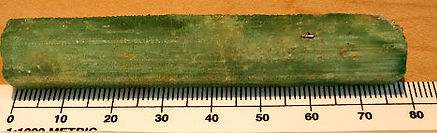
Green beryl, Hedenite, North Carolina
Other Lines of Research - Future Projects and/or for Interested Students
1) The evolution and P-T-t paths of aluminous granulites associated with Proterozoic massive sulfide deposits.
Earlier studies on metamorphic terranes in the Front Range, Colorado, focused on mineral reactions in aluminous granulites to determine the P-T path followed by the rocks (Heimann et al. 2006, Berke et al. 2022). This will be expanded to other locations already sampled in the Rocky Mountains and the Curnamona Province of Australia, and will include age dating to quantify time scales of metamorphic processes.
Garnet crystals exhibiting unusual textures in 1.69 Ga garnet-rich rocks spatially associated with metamorphosed metallic mineralization, Thunderome, Curnamona Province, Australia.


2) Geochemistry and genesis of garnet- and gahnite-quartz rocks from the Nine Mile Pb-Zn occurrence, Curnamona Province, Australia. This is a project in collaboration with Mr. Wolfgang Leyh (Eaglehawk Geological Consulting, Pty., NSW, Australia). MS student Erica Serna worked on this project. Funded by Thomas Harriot College of Arts and Sciences and Research and Graduate Studies of East Carolina University , and Sigma Xi.
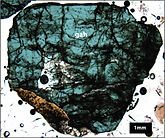
3) Developing gahnite as a petrogenetic and metalogenetic mineral indicator for rare-metal (critical minerals) mineralization in granitic pegmatites using major- and trace-element compositions. This project aims at investigating the composition of gahnite in various kinds of granitic pegmatites worldwide to determine pegmatitic melt evolution and the genesis of rare metal mineralization. MS student Jason Yonts worked on this project. Funding so far from the U.S.G.S. Mineral Resources External Research Program, East Carolina University, Sigma Xi, and the Society of Economic Geologists.

4) Developing garnet as a petrogenetic and metalogenetic mineral indicator for rare-metal mineralization in granitic pegmatites using major- and trace-element compositions. This project aims at investigating the composition of garnet in various kinds of granitic pegmatites worldwide to understand pegmatitic melt evolution and the genesis of rare-metal mineralization, particularly Li. The picture to the left is of a garnet 'line rock' sample from the Pala Chief pegmatite-aplite composite dike, San Diego County, California, generously provided by Dr. David London. Funding is from the U.S.G.S. Mineral Resources External Research Program, East Carolina University, Sigma Xi, and the Society of Economic Geologists. MS student Nicholas Mitchell worked on this project.
Garnet 'line rock', Pala Chief pegmatite-aplite composite dike, San Diego County, California. Generously provided by Dr. David London
5) Identification and characterization of Precambrian microfossils in marine environments in slightly metamorphosed 1.69 Ga marine sedimentary sequences associated with metallic (Pb-Zn-Ag, Cu-Au) mineralization in the Curnamona Province, Australia, and their relationship to minerallization processes. This is a continuation of previous studies in which we characterized the geochemistry of metasedimentary sequences from the southern Curnamona Province, Australia (Heimann et al. 2013) and detected the occurrence of organic matter and likely microfossils, including stromatolites and unicellular organisms, spatially associated with sulfide mineralization, which will be the subject of future studies. The goal is to determine the relationship between the occurrence of marine microorganisms and the formation of specific minerals and mineralization in a marine environment associated with evaporites and carbonates.